Sae-Hun Park, PhD
In humans, about 25,000 different proteins are responsible for most aspects of biological function. To attain their functionality, the majority of proteins must fold into well-defined three-dimensional structures and maintain their structural stability and flexibility throughout their lifetime. However, nascent proteins synthesized inside the cell are constantly at risk of attaining non-native conformations that prevent them from functioning properly and/or cause them to aggregate. Therefore, cells have evolved sophisticated protein quality control (PQC) mechanisms to regulate the balance between production and elimination of misfolded proteins, ensuring proteomic integrity.
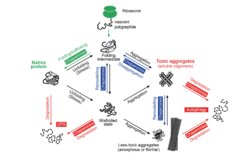
To circumvent protein misfolding problems, cells have developed robust and interconnected compartmental PQC systems. These systems consist of chaperones, proteolytic pathways, and other accessory proteins. Under stress conditions, molecular chaperones can either assist in refolding misfolded proteins or transfer them to the ubiquitin-proteasome system for degradation. Misfolded proteins that escape these mechanisms tend to accumulate as aggregates, which can be degraded through the autophagy pathway with the help of chaperones. These processes must be tightly regulated to maintain protein homeostasis (proteostasis), as failure of proteostasis control is associated with various age-related neurodegenerative diseases, including Huntington’s, Parkinson’s, Alzheimer’s, and amyotrophic lateral sclerosis (ALS).
Chaperone-Mediated Quality Control and Nuclear Proteostasis in Protein Aggregation
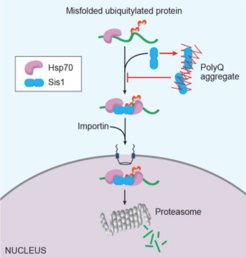
We have reported several important discoveries providing evidence that chaperones participate equally in the folding and degradation of misfolded proteins and that protein aggregates can disrupt cellular waste disposal by sequestering chaperones through the ubiquitin-proteasome system. Furthermore, we are investigating how the nucleus copes with misfolded/aggregation-prone proteins and identifying factors that help maintain nuclear homeostasis. Our studies aim to determine whether and to what extent these fundamental processes contribute to the formation of pathogenic protein plaques in disease contexts.
We also seek to understand how distinct PQC compartments communicate and influence each other using yeast as a model system, an excellent organism for biochemical and genetic analysis. Specifically, we focus on the crosstalk between ribosome-associated quality control (RQC) and other PQC pathways, as well as the biological consequences of RQC deficiencies in proteostasis.
Expanding the Role of Ribosome Quality Control and Mitochondrial Proteostasis

The complexity of PQC mechanisms extends beyond individual compartments, as revealed through our studies on ribosome quality control (RQC). When the RQC pathway fails, stalled nascent polypeptides that would normally be degraded accumulate as detergent-resistant aggregates, leading to proteotoxic stress. These aggregates sequester cytosolic chaperones, interfering with general PQC pathways and potentially contributing to neurodegenerative conditions.
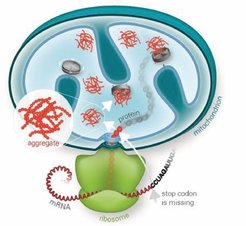
Further investigations into the interplay between RQC and mitochondrial homeostasis have revealed that stalled polypeptides escaping cytosolic degradation are often imported into mitochondria, where they pose a significant risk to proteostasis. The cytosolic protein Vms1 plays a crucial role in mitigating this process by antagonizing Rqc2-mediated CAT tailing, thereby preventing aggregate formation and ensuring that aberrant polypeptides are directed toward mitochondrial degradation pathways. These findings highlight a specialized rescue pathway, mitochondrial RQC (mitoRQC), which is essential for maintaining mitochondrial function under conditions of respiratory stress.
New Insights into Stress-Dependent Phase Separation
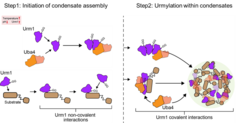
Recent research has uncovered an unexpected role for Urm1, a ubiquitin-related modifier, in stress-dependent phase separation and condensate formation. Cellular stress triggers an increase in Urm1 levels, leading to the covalent modification of target proteins and their sequestration into stress granules and nuclear condensates. Urm1 exhibits self-association upon intracellular acidification and co-condenses with its conjugating enzyme Uba4, forming reaction centers that facilitate urmylation. Loss of Urm1 disrupts condensate formation and compromises stress resilience, revealing a new mechanism by which cells dynamically regulate protein quality control under challenging conditions.
By integrating biochemical, genetic, and cell biological approaches, our research continues to uncover the molecular mechanisms underlying protein homeostasis, stress adaptation, and disease pathogenesis.
People involved in the work
Lucas Cairo, PhD (Former employee)
Chandhuru Jagadeesan, PhD